Molecular Imaging-Informed Nanoengineering
PI: Girgis Obaid, PhD, Assistant Professor of Bioengineering at UT Dallas
Despite tremendous strides in the advancement of sophisticated ligand-directed nanoparticles, they often yield inconsistent results when translocating as an extension from in vitro success to a complex in vivo environment. This is largely due to difficulties in defining molecular specificity at the cellular level in vivo, irrespective of global tumor uptake that can oftentimes be skewed by the dominating pharmacokinetics and the enhanced permeability and retention (EPR) effect within the tumor. In this project, quantitative dual-tracer molecular binding imaging is used as the primary criterion for defining in vivo molecular selectivity of modular nanoconjugates at the cellular level, and will thus accurately direct their tailored bioengineering to enhance outcomes of heterogeneously-targeted head and neck cancer phototherapies.
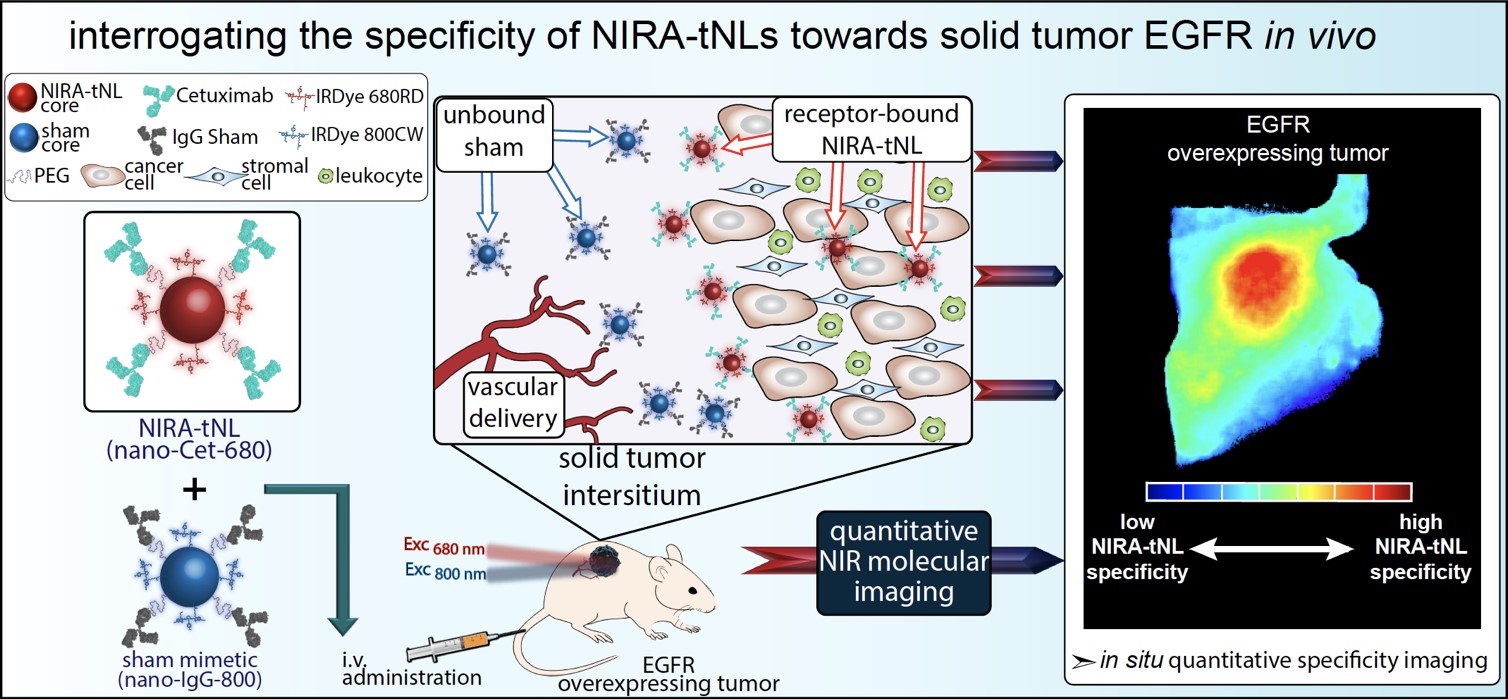
Tumor activatable probes for post-surgical resection phototherapy
PI: Girgis Obaid, PhD, Assistant Professor of Bioengineering at UT Dallas
Fluorescent therapeutic antibodies are emerging as effective molecular probes for image guided surgery. However, the extraneous interstitial presence of non-specifically accumulated probe gives rise to false-positive detection of tumor tissue and margins. The need for molecular resolution is accentuated during microsurgical resection of disease tissue in sensitive anatomical sites. Thus, tumor-cell activation provides a superior mechanism of delineating tumor margins and small tumor deposits. Combining molecular targeting with intracellular activation circumvents the presence of extracellular, non-specific signals of targeted probe accumulation. This project develops a timely approach for enhancing the imaging specificity of clinical antibody-based activatable molecular nanoprobes. This dual-activatable nanoprobe approach is anticipated to enhance the specificity of imaging for image guided surgery. This tunable platform technology can be extendable to various antibodies, fluorophores, nanoprobes and disease indications with the ultimate goal of leveraging photodynamic therapy for the eradication of unresectable disease.
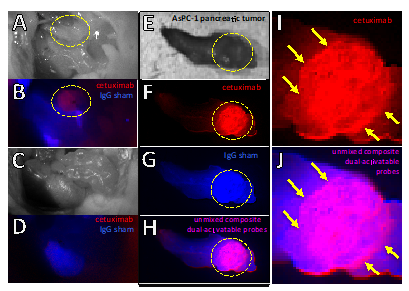
Cancer Mechanics and Remodeling
PI: Jacopo Ferruzzi, PhD, Assistant Professor of Bioengineering at UT Dallas
The mechanical properties of soft biological tissues are implicated in essential biological processes, such as development and wound healing, as well in the pathogenesis of many human conditions including cancer, cardiovascular disease, and neurological disorders. The biomechanical function of tissues evolves through remodeling, a process involving local extracellular matrix (ECM) deposition, degradation, and physical engagement by cells. To better understand the complex roles played by tissue remodeling in normal and diseased conditions, accurate quantification of 3D tissue mechanics is of the essence, though not currently possible with high spatial and temporal resolution. We seek to address this unmet need by establishing a new micro-rheological approach for measuring spatio-temporal changes in ECM mechanics due to cell-driven remodeling. We propose to use an active technique based on magnetic twisting cytometry to map the evolving mechanics of 3D biological tissues. We seek to validate our approach by using breast cancer spheroids embedded in collagen gels as an in vitro model system where heterogeneous ECM remodeling occurs during invasion. Magnetic twisting will be combined with confocal microscopy to monitor evolving structural and mechanical properties of collagen at multiple locations within our gels and over multiple days of culture. Finite element analyses based on experimental data will be used to measure, for the first time, how tumor spheroids alter ECM mechanics during invasion.
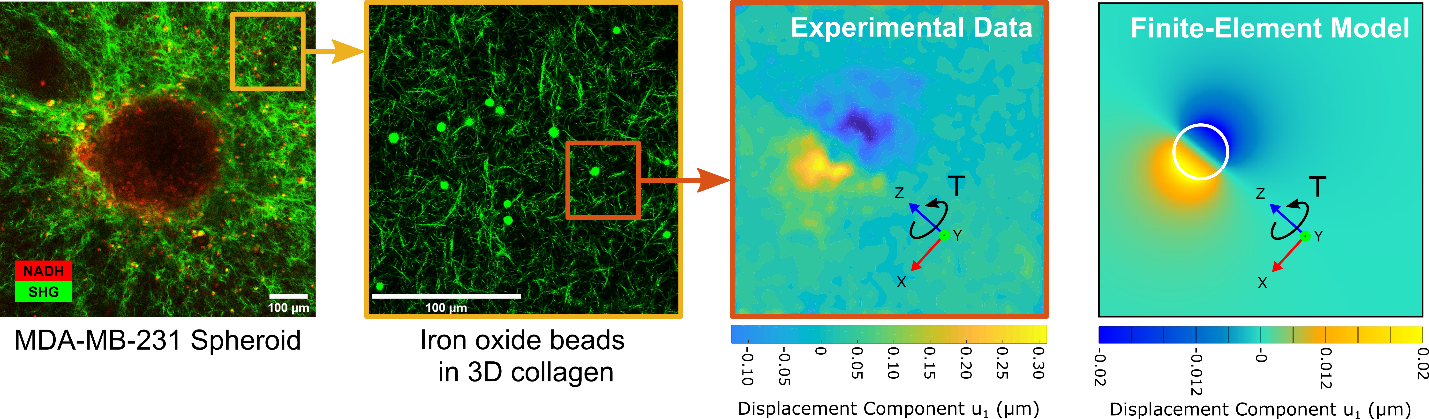
Arterial Stiffening
PI: Jacopo Ferruzzi, PhD, Assistant Professor of Bioengineering at UT Dallas
Arterial aging leads to central artery stiffening by means of marked changes in artery geometry, composition, mechanical properties, and associated function. These local changes manifest themselves via global hemodynamic effects that increase the workload on the heart and cause microvascular damage to organs such as the brain and kidneys. We are developing combined in vivo and ex vivo protocols to investigate the progression of arterial stiffening in experimental animals undergoing normal and accelerated aging to improve fundamental understanding of the underlying degenerative processes. (In collaboration with Jay D. Humphrey (Yale University) and C. Alberto Figueroa (University of Michigan))
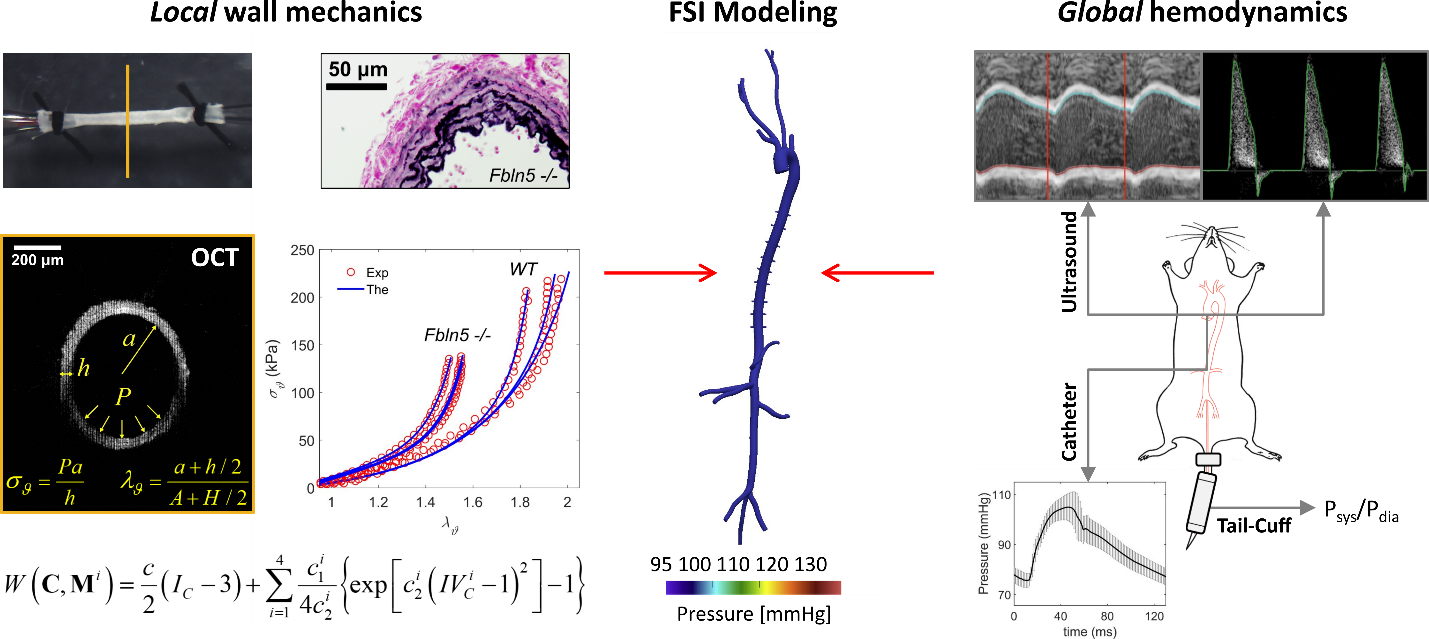
Hyperspectral Imaging (HSI)
PI: Baowei Fei, PhD, EngD, Professor of Bioengineering at UT Dallas and Professor of Radiology at UT Southwestern Medical Center
Hyperspectral imaging (HSI) also called imaging spectrometer is an emerging imaging modality for medical applications. HSI has been explored for various remote sensing applications by NASA. With the advantages of acquiring two dimensional images across a wide range of electromagnetic spectrum, HSI has been applied to archaeology and art conservation, vegetation and water resource control, food quality and safety control, forensic medicine, crime scene detection, and biomedical areas, etc.
HSI offers great potential for non-invasive disease diagnosis and surgical guidance. Light delivered to the biological tissue undergoes multiple scattering from inhomogeneity of biological structures and absorption primarily in hemoglobin, melanin and water as it propagates through tissue. It is assumed that the absorption, fluorescence and scattering characteristics of tissue change during the progression of disease, therefore the reflected, fluorescent and transmitted light from tissue captured by HSI carries quantitative diagnostic information about tissue pathology. In recent years, the advancements of hyperspectral cameras, image analysis methods and computational power make it possible for many exciting applications in the medical field.
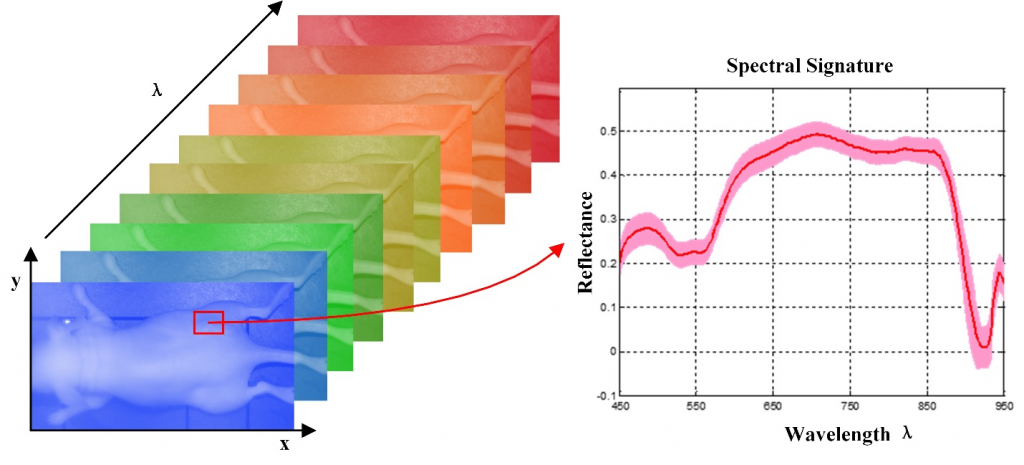
Cardiac Imaging
PI: Baowei Fei, PhD, EngD, Professor of Bioengineering at UT Dallas and Professor of Radiology at UT Southwestern Medical Center
Heart failure affects ≥5 million people in the USA. About half the people who develop heart failure die within 5 years of diagnosis. It is a leading cause of hospitalization, death, and disability in developed countries, creating a significant social and economic burden for patients and health care systems. The treatment costs are estimated to be $32 billion each year. While the underlying cause of heart failure may be quite varied, common features of the failing heart include myocyte hypertrophy and fibrosis. Currently, most methods for the detection of heart failure focus on geometric abnormalities utilizing MRI, ultrasound and CT. Although there have been tremendous improvements in diagnostics for cardiac disease, many of the current techniques are useful only when significant cardiac dysfunction is present. It is well documented that early detection can rescue patients from acute failure and save lives. Early detection of ventricular dysfunction, before progression to full heart failure, is essential to improve patient outcomes.
Cardiac fibers directly affect the mechanical and physiological properties of the heart. During the cardiac cycle from systole to diastole, cardiac fibers are the basic units that pump blood from the ventricles into circulation. Cardiac fiber orientation plays an important role in determining the stress distribution within the myocardial walls. Abnormal cardiac fiber orientations in heart failure patients adversely impact cardiac function and also contribute to arrhythmias that can lead to sudden death. Mapping cardiac fiber orientation enables monitoring of the development and progression of heart failure and provides insight into the mechanism of heart failure for early detection. It is reported that cardiac fiber angles changes through the wall from epicardium to endocardium and that the angles increase from systole to diastole. In a normal heart, the change of fiber orientation is from around -75° at the epicardium to +75° at the endocardium.
Alterations of cardiac fiber orientation can have significant pathological consequences. Animal studies have shown that ventricular transmural fiber rotation was significantly changed in hypertrophic versus normal hearts and that muscle fiber stress and strain across the ventricular wall are very sensitive to the transmural distribution of helix angle. During heart beating, the fiber angles in systole are increased through the wall relative to their counterpart in diastole.
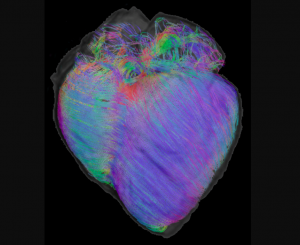
Augmented Reality for Image-Guided Interventions
PI: Baowei Fei, PhD, EngD, Professor of Bioengineering at UT Dallas and Professor of Radiology at UT Southwestern Medical Center
We are developing an augmented reality (AR) system for the integration of previously acquired radiological images (e.g., CT or MRI) with intraoperative ultrasound scans for guiding interventional procedures in real time. Our first application is AR-guided renal biopsy. We have designed and developed an AR system to guide a biopsy needle for improving the accuracy of lesion targeting. In this system, holographic lesion representations are displayed in its real-world location based on the segmentation label provided on the preoperative CT scans. The lesion hologram is then transformed based on the intraoperative deformation and movement observed through real-time ultrasound imaging, removing the need for the surgeon to register the images mentally. A magnetic tracker attached to the biopsy needle allowed the system to predict the needle trajectories that would accurately target the lesion, thereby offering potential improvements in diagnostic specificity. We also designed and developed kidney phantoms to evaluate the AR system performance for lesion targeting.
During the biopsy procedure, the clinician wore the augmented reality headset which displays holographic representations of the lesions, based on the CT scans taken. The clinician uses an ultrasound transducer to gather real-time images. A magnetic tracking is used to determine locations of the ultrasound transducer and biopsy needle, making it possible to display holographic representations of the ultrasound scans and the trajectory of the needle.
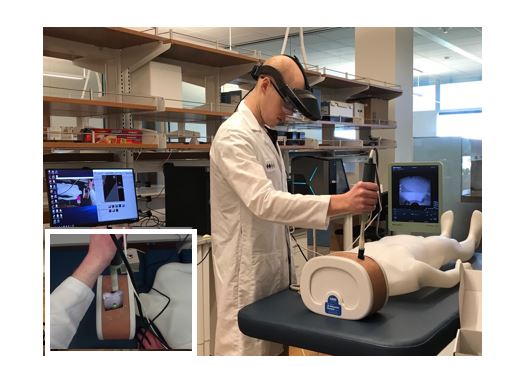
Deep Learning-Based Framework for In Vivo Identification of Glioblastoma Tumor during Brain Surgery
PI: Baowei Fei, PhD, EngD, Professor of Bioengineering at UT Dallas and Professor of Radiology at UT Southwestern Medical Center
The main goal of brain cancer surgery is to perform an accurate resection of the tumor, preserving as much normal brain tissue as possible for the patient. The development of a non-contact and label-free method to provide reliable support for tumor resection in real-time during neurosurgical procedures is a current clinical need. Hyperspectral imaging is a non-contact, non-ionizing, and label-free imaging modality that can assist surgeons during this challenging task without using any contrast agent. We are developing a deep learning-based framework for processing hyperspectral images of in vivo human brain tissue. The proposed framework was evaluated by our human image database, which includes 26 in vivo hyperspectral cubes from 16 different patients, among which 258,810 pixels were labeled. The proposed framework is able to generate a thematic map where the parenchymal area of the brain is delineated and the location of the tumor is identified, providing guidance to the operating surgeon for a successful and precise tumor resection. The deep learning pipeline achieves an overall accuracy of 80% for multiclass classification, improving the results obtained with traditional support vector machine (SVM)-based approaches. In addition, an aid visualization system is presented, where the final thematic map can be adjusted by the operating surgeon to find the optimal classification threshold for the current situation during the surgical procedure.
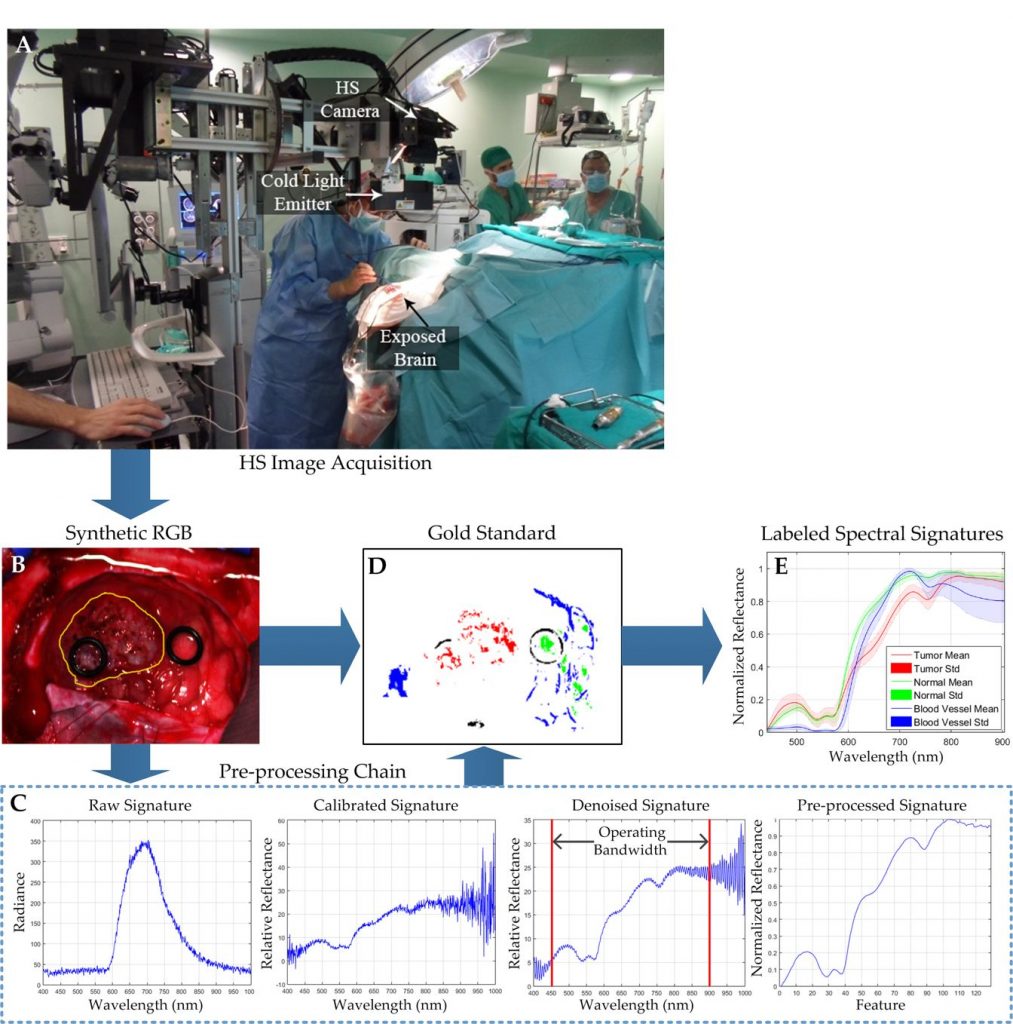
Fabelo H, Halicek M, Ortega S, Shahedi M, Szolna A, Piñeiro JF, Sosa C, O’Shanahan AJ, Bisshopp S, Espino C, Márquez M, Hernández M, Carrera D, Morera J, Callico GM, Sarmiento R, Fei B. Deep Learning-Based Framework for In Vivo Identification of Glioblastoma Tumor using Hyperspectral Images of Human Brain. Sensors (Basel). 2019 Feb 22;19(4). pii: E920.
Perfusion-Guided Monitoring of Tumor Response to Sonopermeabilization: A Novel Strategy for Monitoring and Predicting Liposomal Doxorubicin Uptake In Vivo
PI: Shashank Sirsi, Ph.D., Assistant Professor of Bioengineering
The goal of this project is to develop a method of using quantitative ultrasound imaging (qCEUS) as a guide for ultrasound mediated “sonopermeabilization” using microbubble ultrasound contrast agents. Microbubble oscillations in an ultrasound field can permeate tissue and promote extravasation of a wide-variety of circulating therapeutic compounds, however, ways of monitoring this process and predicting drug uptake in vivo are lacking. We have demonstrated that using both 2D and 3D qCEUS imaging to evaluate tumor perfusion kinetics can be an effective way of simultaneously applying and monitoring sonopermeabilization therapy and predicting drug accumulation in vivo.
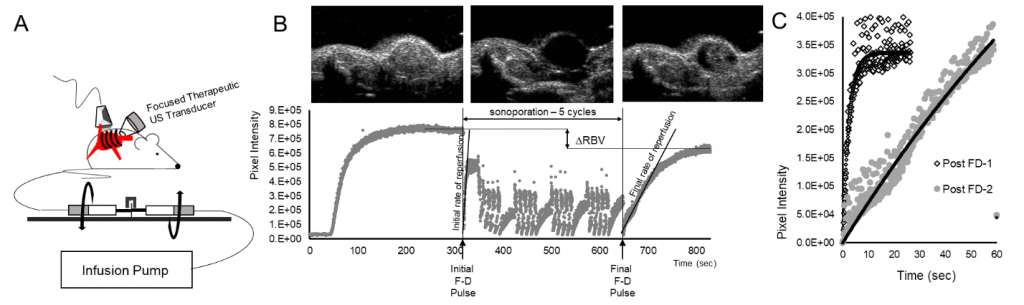
Figure 1 Perfusion-guided imaging of matrigel plug tumor models using qCEUS imaging techniques to monitor sonoporation bioeffects. (A) A rotating syringe pump was developed to administer constant infusions over long periods of time during sonoporation experiments. (B) Screen captures from the clinical ultrasound scanner illustrate non-linear 2D imaging of a neuroblastoma tumor sonoporated using 1×109 MBs. The series of images shows contrast enhancement before sonoporation, followed by focused ultrasound triggered microbubble destruction in vivo during five sonoporation treatment cycles, culminating in MB recovery within the tumor space post-sonoporation; the accompanying time-intensity curve (TIC) has been split into three corresponding stages. (C) MB reperfusion after the initial (300 sec) and final (630 sec) flash-destruction pulses was fitted to an exponential model and the resultant curves were compared to assess sonoporation-mediated changes in perfusion kinetics.
Developing Novel Lipsome-Nanodroplet Acoustic Clusters for Efficient Drug Loading and Release
PI: Shashank Sirsi, Ph.D., Assistant Professor of Bioengineering
Our lab is currently developing novel acoustic biomaterials for advancing the development of image-guided drug delivery platforms in clinical therapies. In this project, we demonstrate a highly efficient method of releasing “caged” small molecule drugs from liposomes in the blood using focused ultrasound application. The drug-loaded liposomes are tethered to vaporizable perfluorocarbon nanodroplets that instantaneously vaporize into gas bubbles upon application of heat and ultrasound. We are demonstrating that the vaporization process can efficiently release small molecule release from the liposomes, which can then diffuse out of the vascular space. This strategy would be highly useful for delivering drugs to tissue, such as the brain, where intact vasculature can prevent drug uptake.
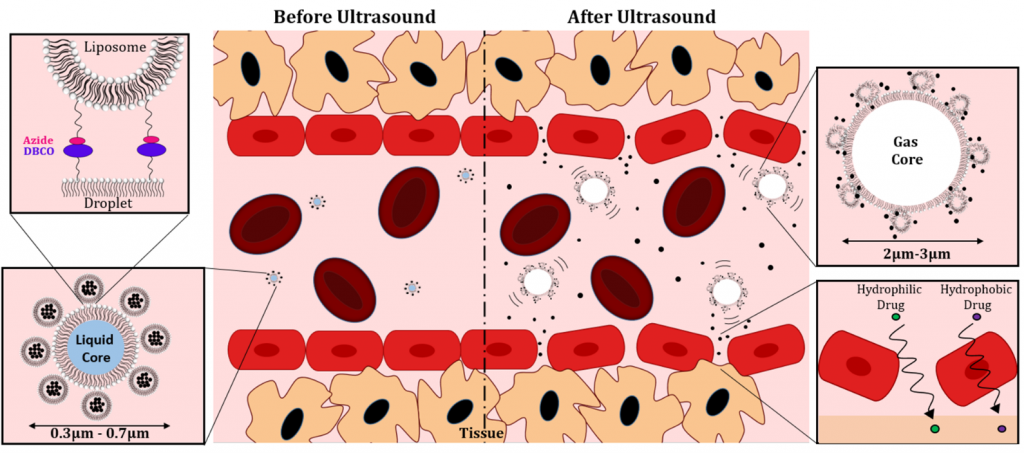
Figure 2. Liposome-Droplet Acoustic Clusters for in vivo drug delivery. Drug-loaded Liposomes are conjugated to the surface of vaporizable perfluorobutane nanodroplets. When exposed to focused ultrasound application, vaporization of the droplets enables rapid release of loaded drugs into the vascular space.
Formulation and Characterization of Chemically Cross-linked Microbubble Clusters
PI: Shashank Sirsi, Ph.D., Assistant Professor of Bioengineering
The purpose of this research project is to introduce a new concept of chemically cross-linked microbubble clusters (CCMCs), which are individual microbubble ultrasound contrast agents (UCAs) physically tethered together. We demonstrate a facile means of their production, characterize their size and stability, and describe how they can potentially be used in biomedical applications. By tethering UCAs together into CCMCs, we propose that novel methods of ultrasound mediated imaging and therapy can be developed through unique inter-bubble interactions in an ultrasound field. One of the major challenges in generating CCMCs is controlling aggregate sizes and maintaining stability against Ostwald Ripening and Coalescence. In this study, we demonstrate that chemically cross-linked microbubble clusters can produce small (<10 μm) quasi-stable complexes that slowly fuse into bubbles with individual gas cores. Furthermore, we demonstrate that this process can be driven with low-intensity ultrasound pulses, enabling a rapid fusion of clusters which could potentially be used to develop novel ultrasound contrast imaging and drug delivery strategies in future studies. The development of novel microbubble clusters presents a simple yet robust process for generating novel UCAs with a design that could allow for more versatility in contrast-enhanced ultrasound (CEUS), molecular imaging, and drug delivery applications. Additionally, microbubble clustering is a unique way to control size, shell, and gas compositions that can be used to study bubble ripening and coalescence in a highly controlled environment or study the behavior of mixed-microbubble populations.
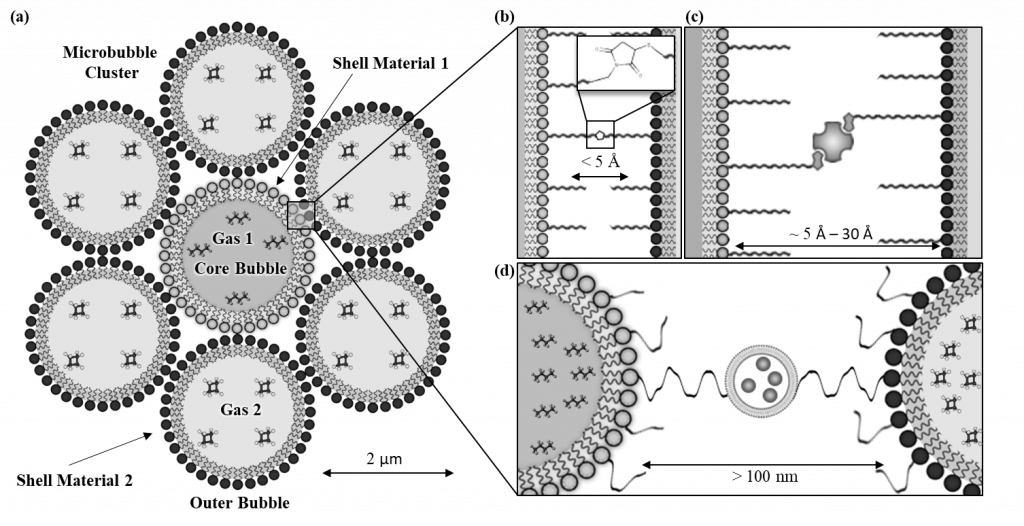
Figure 3. Schematic representation of a microbubble cluster with (a) depicting a microbubble cluster with differing shell material and gas core compositions between the core and outer bubbles. The spacing between the microbubbles within the clusters can be modified by using (b) zero-length cross-linkers (c) protein or polymer interactions and (d) larger molecules such as nanoparticles, microparticles, and vesicles to facilitate the crosslinking.
A Computational Model of the G&R During Atherosclerosis: Integrating Mechanics and Biology
PI: Heather Hayenga, Ph.D., Assistant Professor of Bioengineering at UT Dallas
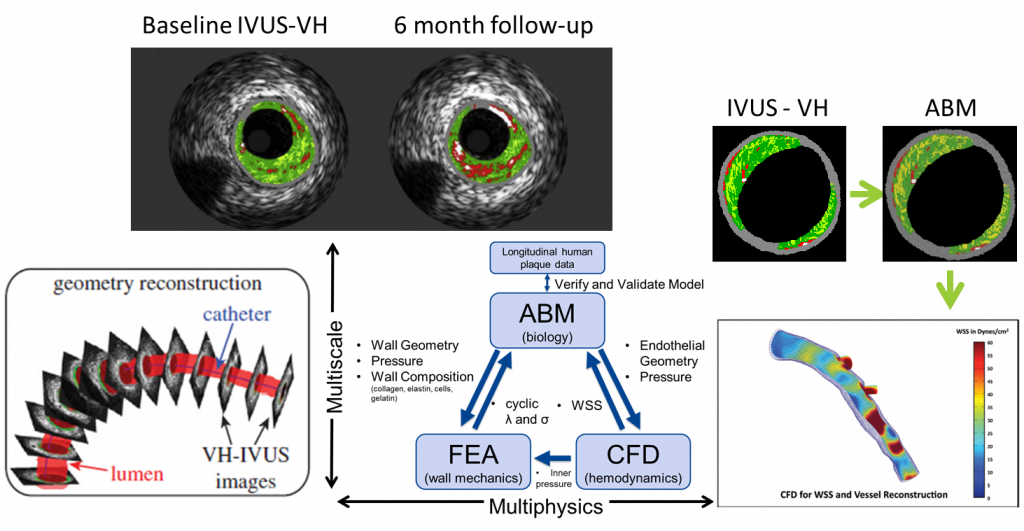
The Vascular Mechanobiology Laboratory at UT Dallas is creating a comprehensive computational model capable of describing the mechanical and biological properties of an atherosclerotic artery as it remodels. The model uses patient specific vascular imaging (IVUS-VH, angiogram, and Doppler) in order to reconstruct the geometry in the Agent Based Model (ABM). It then uses Finite Element Analysis (FEA) and Computational Fluid Dynamics (CFD) to calculate the wall mechanics and blood hemodynamics, respectively, of the artery. Together the ABM-FEA-CFD model is capable of determining the biological and mechanical factors of the artery at the current state and predict how the artery will remodel over time. This model will enable clinicians and patients to ability to quickly determine vessel vulnerability, better understand underlying mechanisms of plaque growth, as well as predict optimal treatment plans and outcomes.
Targeted Neuroplasticity Lab
PI: Seth Hays, Ph.D., Assistant Professor of Bioengineering at UT Dallas Director of Preclinical Research for the Texas Biomedical Device Center
The targeted neuroplasticity lab employs elements of neuroscience and biomedical engineering to develop treatments for human disease. The primary research focus of the lab is to develop new strategies to enhance neuroplasticity, or the ability of the brain to change, in order to treat neurological disease. The majority of current studies evaluate the ability of vagus nerve stimulation (VNS), a putative targeted plasticity therapy, to improve recovery of motor dysfunction. We are rigorously testing VNS to enhance recovery in clinically-relevant animal models of stroke, peripheral neuropathy, and spinal cord injury. In addition to further development and optimization of targeted plasticity therapy, we are investigating the cellular and molecular mechanisms that underlie this recovery. Finally, building on our success in animal models, we are in the process of translating VNS-based targeted plasticity therapy to clinical investigation in patients with chronic stroke and spinal cord injury.
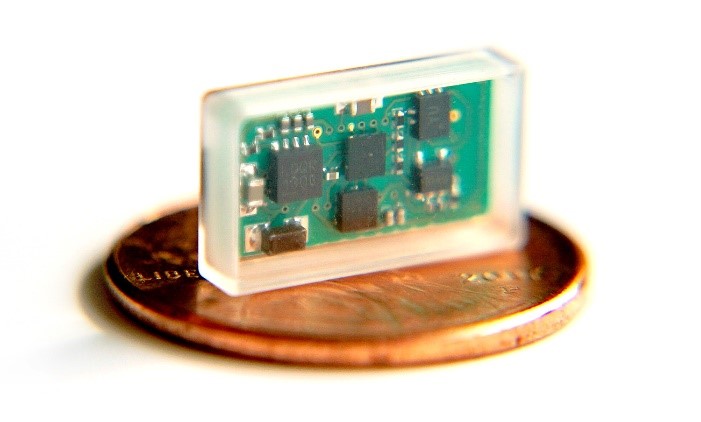
Miniature VNS device
Currently available implantable devices for vagus nerve stimulation have technical limitations that may limit their utility. These include a large profile that necessitates two incisions and tunneling during implantation, fragility of the electrode leads, and presence of an on-board battery that must be surgically replaced. We are developing and optimizing a novel miniature, wireless, battery-free vagus nerve stimulation device to obviate these issues. The device is undergoing bench and preclinical evaluation to examine nerve recruitment. Clinical trials using the device are expected to begin in 2020.
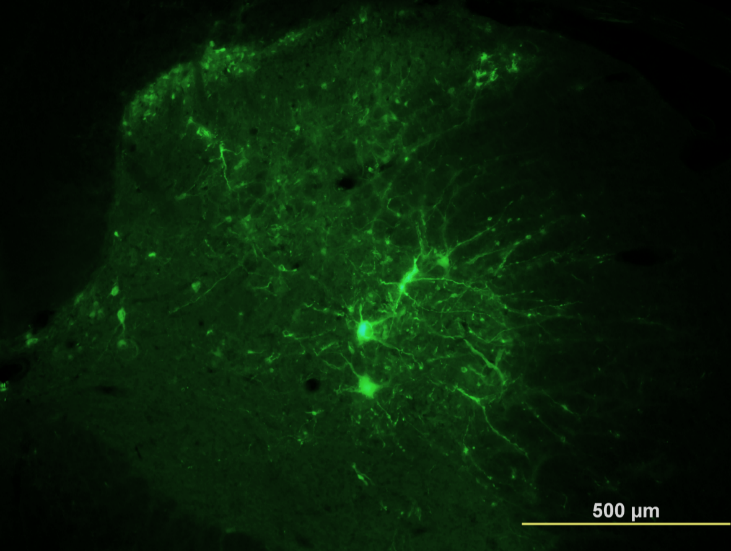
Labeled neurons in the spinal network indicating enhanced synaptic connectivity after VNS therapy
Nanosurgery by Molecular hyperthermia
PI: Zhenpeng Qin, Ph.D., Assistant Professor of Mechanical Engineering
Precise manipulation of protein activity in living systems has broad applications in biomedical sciences. However, it is challenging to use light to manipulate protein activity in living systems without genetic modification. Here, we have developed a new method to optically and precisely switch-off protein activity in living cells without genetic modification by olecular hyperthermia (MH), based on nanoscale plasmonic heating of gold nanoparticle (AuNP). We have shown that targeted membrane receptors such as protease activated receptor 2 (PAR2), a G-protein coupled receptor, can be photo-inactivated in situ by MH without compromising cell proliferation. Furthermore, MH gives molecular specificity for photo-inactivation of targeted receptors while leaving other receptors intact. The newly developed MH enables selective and remote manipulation of protein activity and cellular behavior without genetic modification.
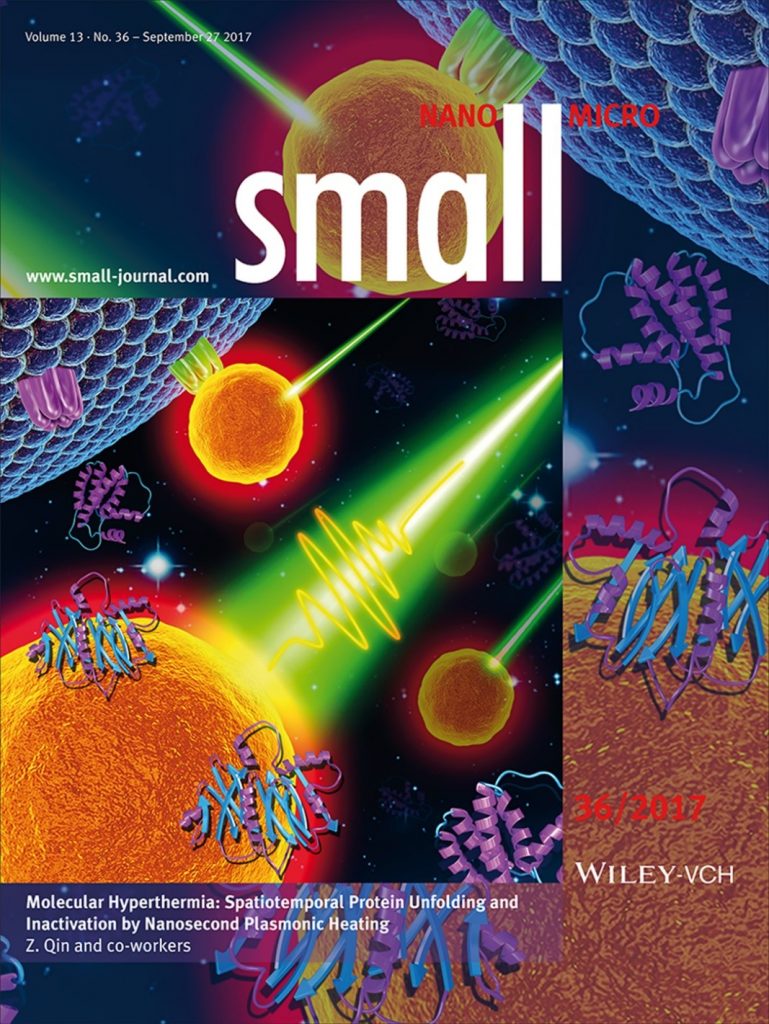
Optical microscopy
PI: Yichen Ding, Assistant Professor of Bioengineering, UTD
Our light-sheet fluorescence microscopy (LSFM) for rapid data acquisition, high spatiotemporal resolution, and minimal photobleaching, demonstrates the capacity for scanning a region of interest with specific fluorescence contrast. This cutting-edge imaging technique allows for elucidating the differentiation of stem cells in cardiac regeneration, providing an entry point to discover novel micro-circulation phenomenon with clinical significance for injury and repair.
Mixed reality-based interactive quantification
PI: Yichen Ding, Assistant Professor of Bioengineering, UTD
We interface the virtual environment with imaging data to navigate through the microenvironments with a user-directed perspective for interactively studying cardiac mechanics, physiology, and developmental biology. This framework is compatible with a low processing power smartphone equipped with a headset, leading to a pipeline for developing an interactive virtual laboratory for life science investigation and learning.
Displacement analysis
PI: Yichen Ding, Assistant Professor of Bioengineering, UTD
We establish a vector field to assess myocardial deformation in response to cardiac injury. This method enables us to investigate 4D cardiac contractile function in segmental myocardium, and to map the focal cardiac mechanics under physiological and pathological conditions. We also apply this method for the analysis of the heartbeat-mediated pulsatile displacement in blood vessels, providing a quantitative perspective to interpret the large volume of imaging data.